Millions saw the apple fall, but Newton asked why.
Why don't birds get an electric shock when they sit on a live wire?
Education is not about teaching kids how to read, but about teaching them to question what they read. We guide children how to think, not what to think.
Education is not about teaching kids how to read, but about teaching them to question what they read. We guide children how to think, not what to think.
Download our App to check out our unmatched quality of questions.
Why don't birds get an electric shock when they sit on a live wire?
Traditional Teaching
Teacher teaches in class
Student memorizes the definition and formula
Student passes the exam but the underlying concepts are still not clear
Traditional teaching is “one size fits all”, whereas every child is different. Rather than student-focussed learning, the currently established methods of teaching rely on standards, curriculum and passing tests.
How we help students?
Teacher teaches in class
Mind Actuators empowers students to ask thought provoking questions
Actuate Test probes students on the thorough understanding of concepts
Advanced analytics identifies learning gaps and communicates to teachers and parents
Personalised follow up and Reactuate Test for every student to address the gaps
Mind Actuators empowers students to ask thought provoking questions
Actuate Test probes students on the thorough understanding of concepts
Advanced analytics identifies learning gaps and communicates to teachers and parents
Personalised follow up and Reactuate Test for every student to address the gaps
Clear concepts allow students to excel in their exams
Our approach is uniquely tailored considering the different needs of every student. At the center of our approach is the student, and value is built around the questions a student needs answers to, in order to benefit from the curriculum.
Who we are?
We are a group of education enthusiasts with a vision to help students realise their true potential by means of a journey guided by conceptual learning. We strive to make use of modern technological enhancements coupled with effective educational techniques to deliver the highest value to a child’s learning journey.
Our team consists of expert professionals who are former graduates from the likes of IITs and NITs, some of India’s finest universities. The group has a strong dynamic personality owing to the diverse mix of individuals brought together by their sheer love for all things maths and science.
Still have some doubts?
What our partners says
Get exclusive app for your school
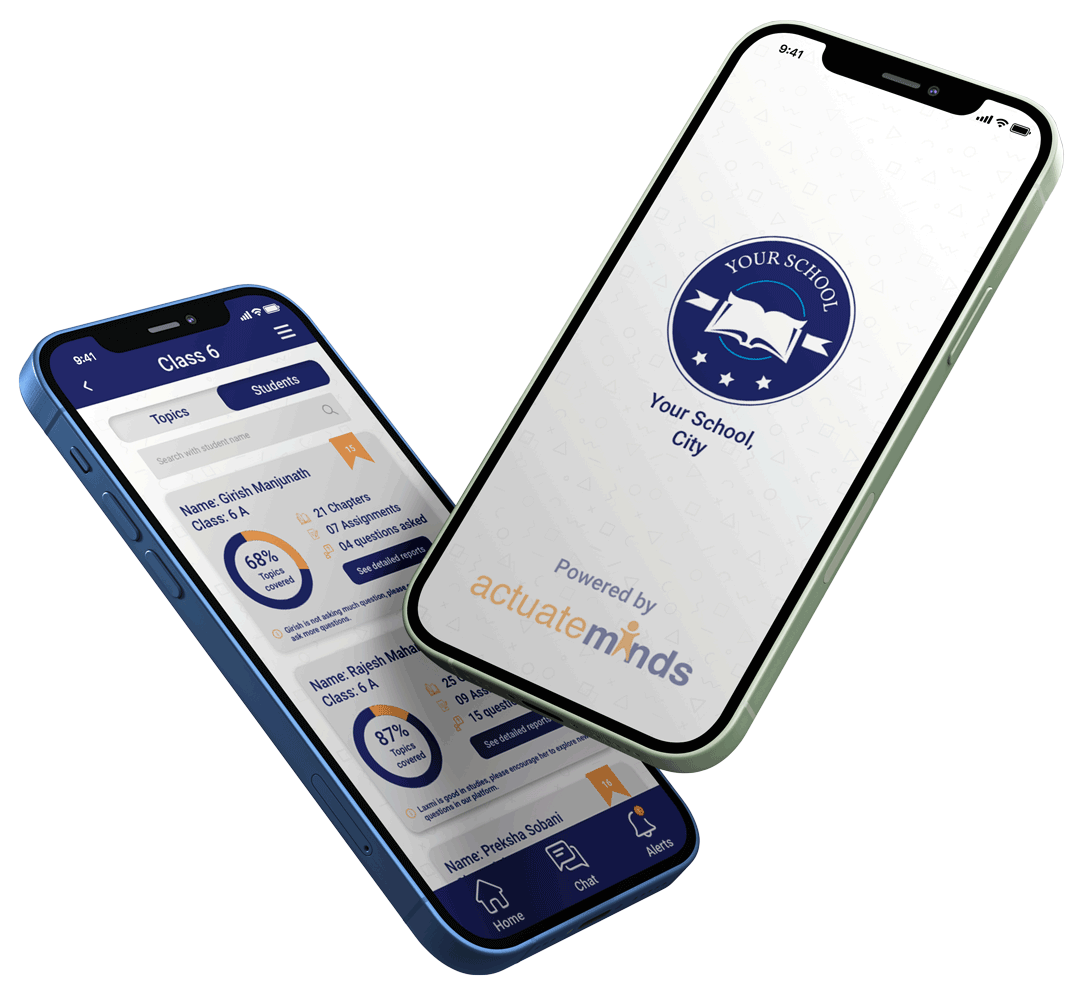
When your school becomes our education partner, we deliver a custom personalised app only for your school.
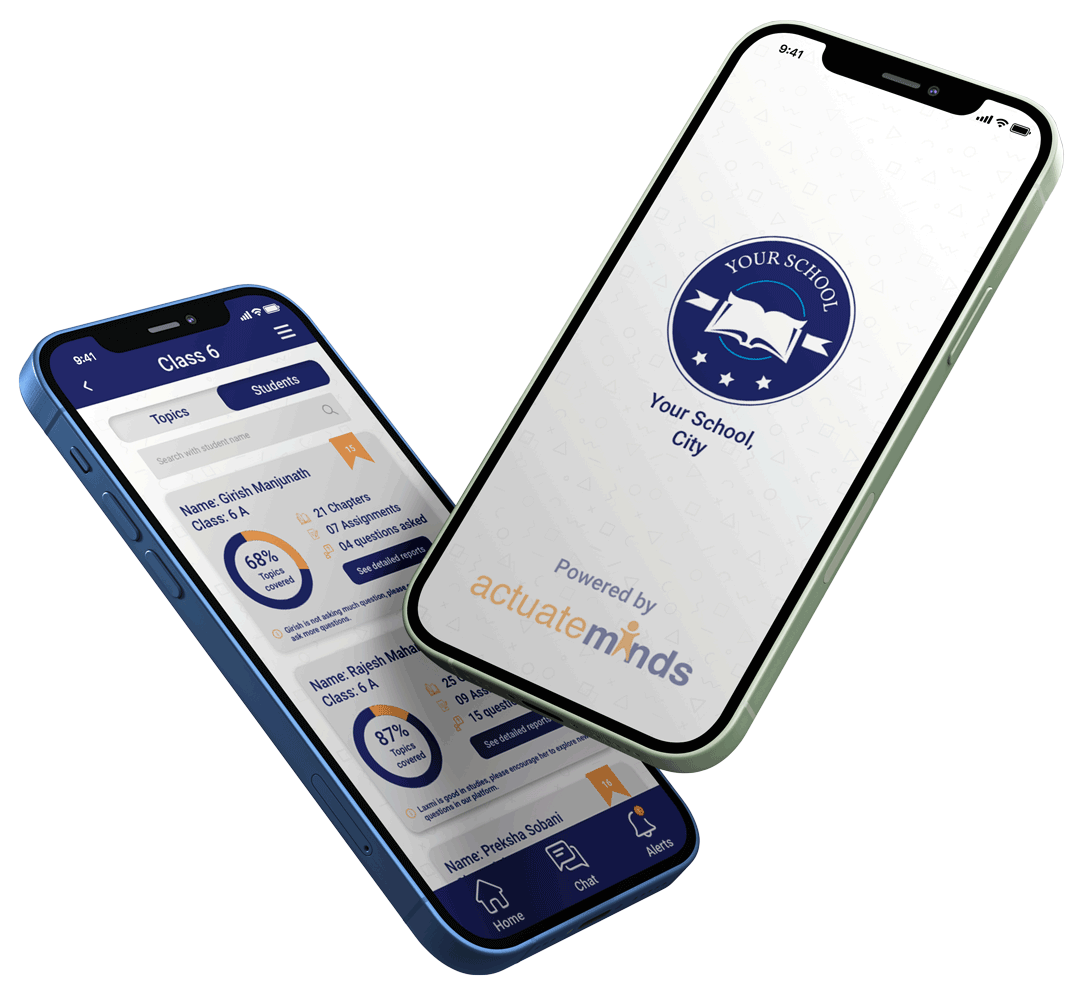